France: Methods for large protein crystal growth for neutron protein crystallography
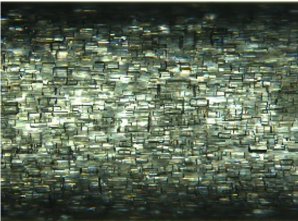
Aligned microcrystals
Neutron protein crystallography can provide crucial information on the location of hydrogen atoms, details relating to hydration, hydrogen bonding, and ligand interactions. This type of information is of direct relevance to academic and pharmacologically-driven research in the life sciences.
The ability to grow large protein crystals is the single biggest bottleneck that limits fuller exploitation of this important approach in structural biology. It was for this reason that macromolecular crystallogenesis has its own work package in SINE2020. For protein crystals to be effectively investigated by neutron crystallography, they need to have volumes in the region of at least 0.1mm3. Theoretically, there is no particular reason why crystals of this size cannot be grown on a fairly routine basis if sufficient effort is invested in the exploration of new approaches.
Biological crystals are usually grown from concentrated protein solutions, with the aid of specific precipitants. In favourable conditions, nucleation occurs, and small crystals emerge from the solution as it concentrates. Key factors in optimizing this process for the growth of larger crystals include the concentration of protein & precipitant (relating to the phase diagram of the protein), the rate of growth, temperature, and the availability of a good supply of protein to sustain growth. Factors that limit or arrest growth are often difficult to identify but sometimes the crystal faces may be obstructed in some way.
The challenge is thus to achieve large crystal growth in a reproducible, time-saving, labour-saving way. It would be ideal if in the future, neutron crystallographers can, after suitable pre-characterisation work, submit their solutions to an automated or semi-automated platform that would allow the exploration of a large range of conditions in a highly systematic way and to allow users to monitor growth from their remote computers.
The Insitute Laue-Langevin (ILL) in Grenoble, France has been investigating new crystal growth methods. The first is the development of a module that could allow larger scale automated approaches in the future (task 1); the second is a flow crystallization system (task 2); the third is the investigation of the the use of high magnetic fields to facilitate the production of larger single crystals – or the alignment of many microcrystals that would then constitute a sample suitable for neutron diffraction.
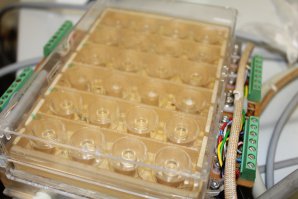
The prototype module with 24 crystallisation wells.
Task 1: A module for automated large crystal growth exploration
This part of the SINE2020 project has focused on the development of a temperature controllable multi-well module in which crystal growth can be optimized. The use of temperature control is one that has been described and demonstrated by Dr. Monika Budayova-Spano and colleagues at the Institut de Biologie Structurale (IBS) in Grenoble.
The idea of designing this module was to scale up the approach so that multiple crystallization wells with individual (programmable) temperature control could be used to explore a wide range of growth conditions. A prototype module was made that consisted of a custom plate design containing 6 × 4 wells where the individual crystallization experiments can occur. Each well can be adapted to different conditions, with each having independent temperature control. The wells are heated using Peltier heating elements with a temperature feedback system that allows each well to be heated and cooled over a temperature range of 4˚C -60˚C, with an accuracy of 0.1 degree. The set-up was designed to allow crystal growth to be monitored and recorded photographically. Crystallization tests have been carried out using trypsin and rubredoxin.
Post SINE2020, the development forseen would be to make these modules “plug and play” so that a more extended ‘robotic’ approach could be used (see Figure 1). Crystallogenesis runs could be removed by the user on completion and other runs installed using another module – the module would be the working unit of a larger array – with all being camera visualisable and providing time-lapse information to a user portal. The schematic diagram shown in Fig. 1 shows how such an array of modules might be configured so as to accommodate a large number of modules that would be easily attachable and removable when crystal growth study had completed.
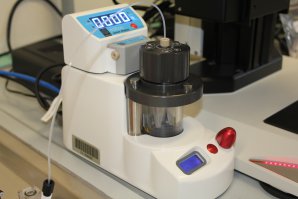
Dolomite Mitos P-Pump
Task 2: Flow crystallization.
Another way of pursuing large crystal growth is the idea of a flow crystallization system. The idea is to maintain steady-state batch conditions around a crystal at all times during its growth, by providing a constant supply of fresh protein stock to the crystallization environment. This will maintain optimal solution conditions at all times and help minimize accumulation of impurities on crystal surfaces – such impurities may hinder crystal growth.
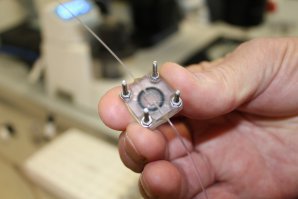
Flow crystallisation chamber
A Dolomite Mitos P-Pump (picture above) was chosen to maintain the extremely low flow rate (between 70-1500 nl min-1) required to regulate the system. A suitable crystallization chamber (picture right) that can connect to the pump was designed and made using a 3D printer. This chamber creates a sealed environment and provides ready access to the crystals once they have grown.
Acknowledgements: Trevor Forsyth and Ashley Jordan, ILL
Back to The Road to the ESS
Other articles: ILL, Crystal Growth, Phase diagram crystal growth, Crystallization Chamber, The use of high magnetic fields for large neutron-sized sample production