Portugal: RPC Results so far
30 November 2018
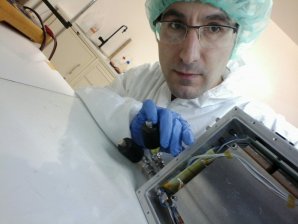
Resistive Plate Chambers are being developed as neutron detectors as part of SINE2020's Detectors work package. You can read more out more about them and how they work in our other articles.
Luís Margato, Andrey Morozov and Alberto Blanco from LIP Coimbra in Portugal have been working on the project and below you can find out what they have been doing.
Step 1: Conceptual design
Luís Margato and his team initially used Monte Carlo simulations to investigate design concepts for 10B RPCs. Using open source codes (ANTS2 toolkit) they assessed the effects of changing the detector parameters and materials: for example the width of the gas gap, converter layer thickness or angle of incidence of the neutron beam on the detector. Once explored, it was time to make some prototypes.
Step 2: Proof-of-concept
As a result of the simulations, a hybrid RPC prototype was constructed at the lab in LIP Coimbra, with the help of C. Hoglund at the ESS detector coatings workshop who was responsible for the production of the coatings. It was tested at ILL in France (as part of an exploratory project funded by FCT). Comparing two RPC prototypes, one with the converter layer and one without, showed that the neutron converter allows neutrons to be detected and at a good spatial resolution too. The concept works!
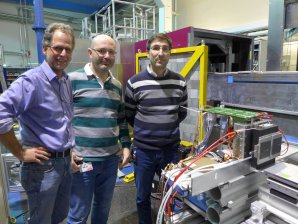
Step 3: Prototypes
Next, two more prototypes with different gas-gap widths (0.35mm and 1mm) were made and tested, in collaboration with Karl Zeitelhack, at FRMII on the TREFF beamline as part of SINE2020. Results showed a spatial resolution better than 0.25mm FWHM and 12.5% detection efficiency for 4.7 angstroms neutrons. These were in good agreement with the MC simulations, including the expected better performance and resolution of the thinner (0.35mm) gas-gap. But can it be improved further by providing several opportunities for neutrons to be captured?
Step 4: Multilayers
Using the better performing gas-gap of 0.35mm, a detector with double-gap RPCs in a multilayer architecture was then designed and assembled at LIP and tested at FRMII. The prototype contained 10 double-gap 10B RPCs (comprising of 20 neutron converter layers) and the spatial resolution performance is maintained as good as for the case of one of the single-gap hybrid RPCs. The measured detection efficiency was about 60% making a multilayer design very encouraging. Both results were again in good agreement with simulations.
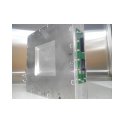
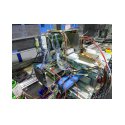
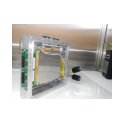
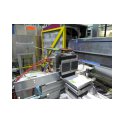
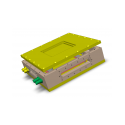
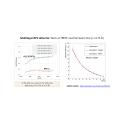
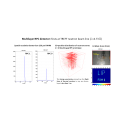
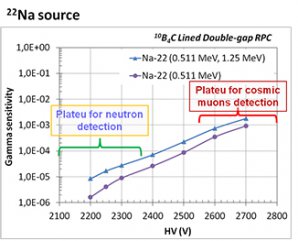
Step 5: Gamma sensitivity
Unfortunately, gamma rays emitted from a sample or by other materials in the neutron beam path can disturb the detectors response contributing false events to the results and so it is important to understand and reduce their effect on the RPCs being developed. Using Co-60 and Na-22 gamma sources, the 10B RPCs are being characterized for their gamma sensitivity. Then when the parameters are evaluated, the design to improve it.
The preliminary results show that for a double-gap RPC, irradiated by an Na-22 gamma source, the sensitivity of the RPC to the Na-22 gamma rays, and in the high voltage (HV) region of the plateau for neutron detection (see figure right), can go down to ~10-6 for the 511keV photons and may go below 10-5 when the 1.27 MeV are taken into account. These results were obtained without any optimization of the detector regarding the gamma sensitivity so by optimizing the detector design concerning this aspect, in principle it will be possible to reduce these values.
Next steps:
With such a promising detector technology in the making we need to work out how to improve the current designs and materials even further, for example optimizing the 10B4C layer thicknesses in the multilayer device to increase the counting rate capability. So for now, Luís is back to the virtual world of simulations using information learned from prototype testing.
In particular, the team are looking at improving the counting rate of the detector, very important as we want these new detectors to count as many neutrons per second per square millimeter as possible.
Other areas for future investigation include modelling of the detector considering neutron scattering in the detector materials and varying the angle of incidence of the neutron beam from the perpendicular incidence.
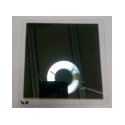
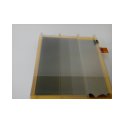
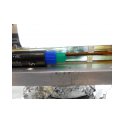
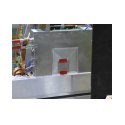
References:
L. M. S. Margato, A. Morozov, Boron-10 lined RPCs for sub-millimeter resolution thermal neutron detectors: Conceptual design and performance considerations
L. M. S. Margato, A. Morozov, A. Blanco, P. Fonte, F. A. F. Fraga, B. Guerard, R. Hall-Wilton, C. Höglund, A. Mangiarotti, L. Robinson, S. Schmidt, K. Zeitelhack
Boron-10 lined RPCs for sub-millimeter resolution thermal neutron detectors: Feasibility study in a thermal neutron beam
Acknowledgements: Luís Margato, LIP
Back to The Road to the ESS
Other articles: Resistive Plate Chambers, RPCs: How they work, LIP, Detectors, MLZ/TUM